Soil Drainage
The use of ABG’s Drainage Geocomposites can save up to 64% carbon footprint compared with traditional granular soil drainage methods:
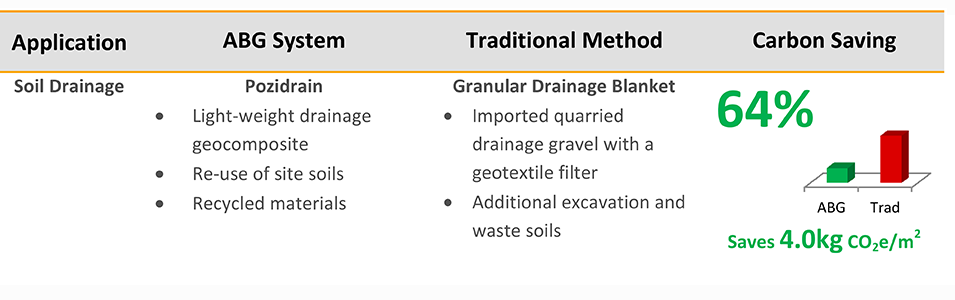
Carbon saving value based on the average value of two assessments: French Drain vs. ABG Fildrain Type 6 (93% carbon saving), and Stone Drainage Blanket vs. Pozidrain 7S250/NW8 (95% carbon saving). The French Drain was assumed to be 700mm deep and 500mm wide, lined with a typical non-woven separation geotextile. Fildrain Type 6 is a 7mm thick drainage geocomposite which is installed vertically in a trench with a perforated pipe at the base. The trench is backfilled with arisings and the system provides equivalent or better drainage performance to standard French Drain designs. It is assumed that the carbon value of installation is approximately equivalent for each method. The Stone Drainage Blanket was assumed to be a 300mm thick layer of drainage gravel lined with a geotextile separator above and below. Pozidrain 7S250/NW8 is a 7mm thick drainage geocomposite which provides equivalent or better water flow to that of a 300mm thick granular drainage layer. The soil sent to waste has not been included in the analysis.
Back of Wall Drainage
The use of ABG Deckdrain geocomposite can save up to 94% carbon footprint compared with traditional concrete block backfilled with gravel methods
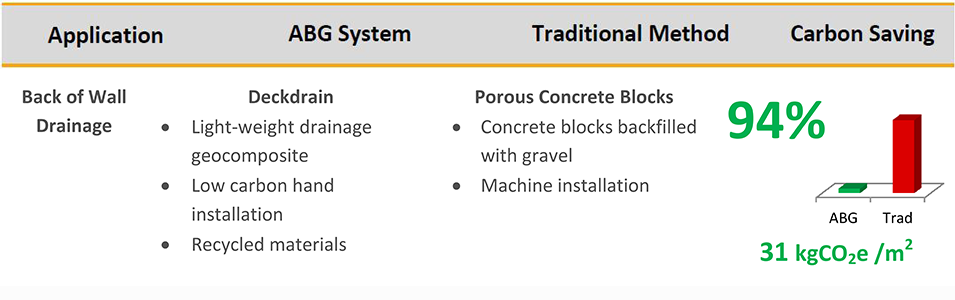
Carbon saving taken directly from the Costain case study (Wilson, 2018) which is a case study comparing the use of ABG Deckdrain with traditional solutions for drainage behind concrete bridge abutment retaining walls. The traditional solutions assessed were no-fines concrete (97% carbon saving) and hollow concrete blocks backfilled with gravel (92% carbon saving). The carbon saving stated is based on the hollow concrete blocks. The assessment in the case study discounted the removal of waste material, was based on actual distances to quarries/suppliers and material quantities as assessed by Costain.
Permeable Paving
The use of ABG Sudspave permeable paving can save up to 54% carbon footprint compared with traditional permeable block paving methods.
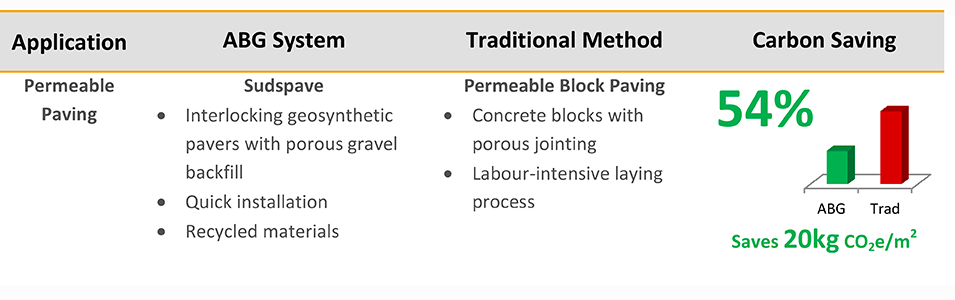
This assessment was based on a permeable block paving surfacing compared with ABG Sudspave – a porous paving surface comprising interlocking plastic pavers backfilled with gravel. The carbon footprint associated with excavation and road foundation construction have not been included in this assessment, since just the surfacing is compared. The carbon footprint associated with construction is also discounted due to the difficulty of comparison, although it is expected that the installation of a Sudspave solution would have a lower carbon footprint. The permeable block paving assumes a 60mm paver on a 50mm bedding layer. The embodied carbon is assessed as 36 kgCO2e/kg (Marshalls.co.uk, 2018) which includes transportation. Added to this is the embodied carbon in the bedding layer and fine gravel in the joints between the pavers. The Sudspave design is a 40mm plastic paver on a 20mm bedding layer (a design which is generally structurally and hydraulically equivalent or better). The carbon footprint of transportation has been added to the Sudspave assessment.
Gravity Retaining Walls
The use of ABG Webwall Geocell can save up to 77% on the carbon footprint compared with traditional gabion basket gravity retaining walls:
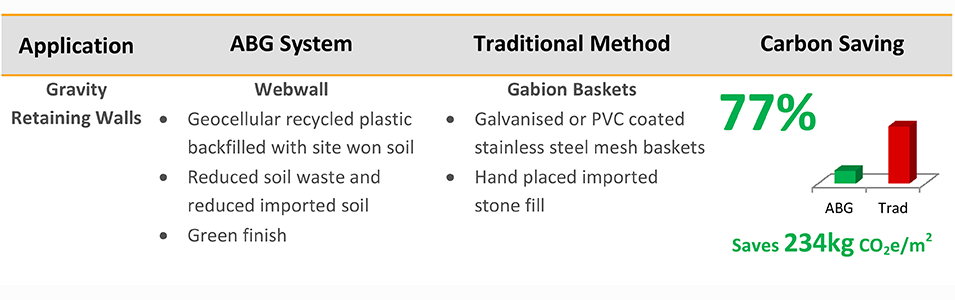
This assessment was based on the results from the ‘Axis Business Park Environmental Bund’ case study from the WRAP report (Corney, 2010). This case study has been adjusted to be specific to the use of ABG products transported to the same location (60 miles from ABG). The WRAP report calculated the carbon footprint of a 9.5m high gabion basket wall and compared it to a that of an equivalent reinforced earth design (the latter was the adopted solution). The ABG carbon saving assessment used the values assessed in the WRAP report for the gabion design and compared it to the calculated footprint of an ABG Reinforced Webwall design very similar to the equivalent reinforced soil design in the WRAP report. The emissions during construction were assumed to be the same as those in the WRAP report.
Highway Foundations
The use of ABG Abgrid geogrid can save up to 21% carbon footprint compared with traditional compacted gravel highway foundation methods.
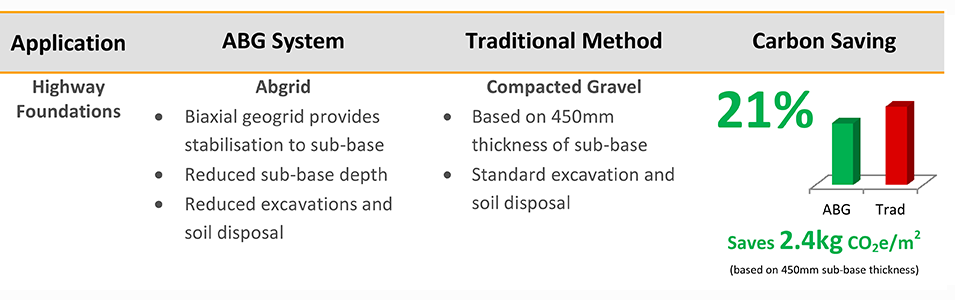
Carbon savings based on a traditional method consisting of 450mm of Type 1 sub-base with a typical non-woven separation geotextile at the base, compared with 300mm of Type 1 sub-base with Abgrid 30/30 (30kN biaxial geogrid) at the base, as well as a typical non-woven separation geotextile. The carbon saving is proportional to the difference between 450mm and 300mm of sub-base considering additional excavation, imported sub-base and construction time, less the additional carbon footprint associated with manufacturing and delivery to site of Abgrid 30/30.
Highway Fin Drains
The use of ABG Fildrain Fin Drain geocomposites can save up to 74% carbon footprint compared with traditional granular stone fin drain methods.
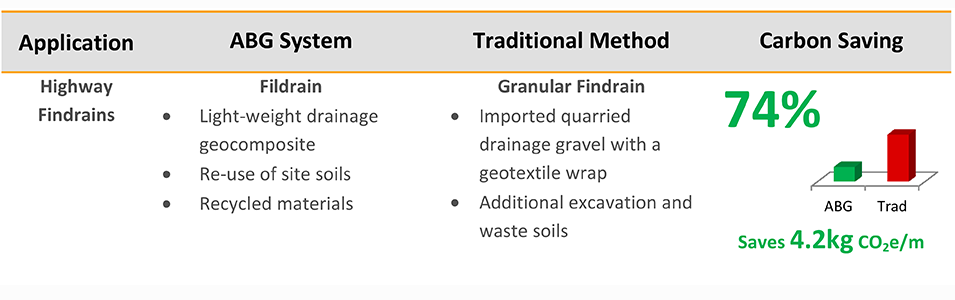
Embankment Starter Layers
The use of ABG’s Fildrain Drainage Geocomposite can save 57% on the carbon footprint compared with traditional granular stone drainage methods for embankment starter layers:
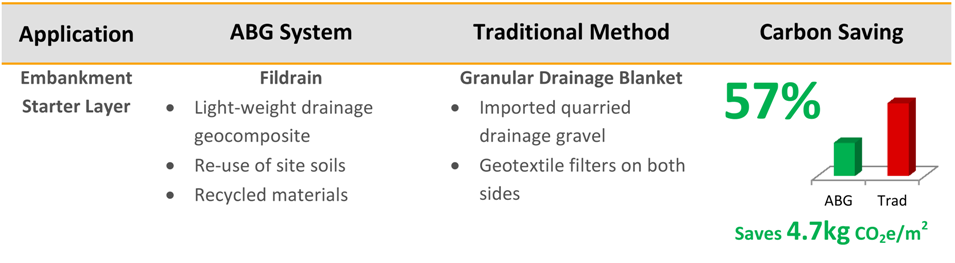
General Assumptions
The analysis method follows that described in the WRAP report (Corney, 2010). The carbon associated with four key stages is assessed a) the carbon associated with waste soil, b) the embodied carbon of imported materials, c) the transportation of imported materials to site, and d) the carbon associated with construction on site. The carbon footprint of waste material is based on fuel burnt during excavation, loading, and transportation to landfill. These calculations assume that waste material is transported at a rate of 10m3 per load, 15 minutes excavation and loading time per load, and a 15 mile return journey to the nearest landfill. The fuel efficiency of the vehicles used is assumes as 25 L/hr (excavation and loading) and 4.4 miles/L (transportation). The carbon footprint of burning diesel is assessed as 2.67 kgCO2e/L based on the value given for ‘Diesel (average biofuel blend)’ in the DEFRA report (Department for Environment Food & Rural Affa irs, 2018).
The embodied carbon of the various imported gravels used in these assessments is assumed to be quarried limestone or similar, with an embodied carbon footprint of 0.09 kgECO2e/kg (kilograms of embodied carbon dioxide equivalent per kilogram of product) as per the ICE report (Hammond and Jones, 2011). The embodied carbon of all ABG’s geosynthetic products is based on ABG internal assessments (Heritage, 2018) and ‘Obtaining reliable embodied carbon values for geosynthetics’ (Raja, 2015).
The transportation of imported materials is generally based on the installation site being 100 miles from ABG and 5 miles from the nearest quarry. Fuel economy is estimated as 4.4 miles/L. The weight of material transported varies for each item. The carbon footprint associated with construction is based on estimates where possible and ignored in more complicated situations for simplicity of calculations.
References
Corney, N. et. al. (2010) Sustainable Geosystems in civil engineering applications, WRAP Geosystems Report MRF116-001
Department for Environment Food & Rural Affairs (2018). UK Government GHG Conversion Factors for Company Reporting.
Hammond, G. & Jones, C. (2011) Inventory of Carbon & Energy (ICE) Version 2.0, Sustainable Energy Research Team (SERT) Department of Mechanical Engineering, University of Bath, UK
Heritage, R. (2018) Embedded Carbon of ABG Geosynthetics, ABG Technical Note [Not yet published]
Marshalls.co.uk (2018) Carbon Footprint Calculator for Hard Landscaping. [online] Available at: https://www.marshalls.co.uk/homeowners/hard-landscaping-carbonfootprint-
calculator [Accessed 18 Sep. 2018].
Raja, J., Dixon, N., Fowmes, G., Frost, M. and Assinder, P. (2015). Obtaining reliable embodied carbon values for geosynthetics. Geosynthetics International.
[http://dx.doi.org/10.1680/gein.15.00020]
Wilson, S. (2018) A14 Deckdrain Back of Wall Drainage, Costain Case Study [Not yet published]